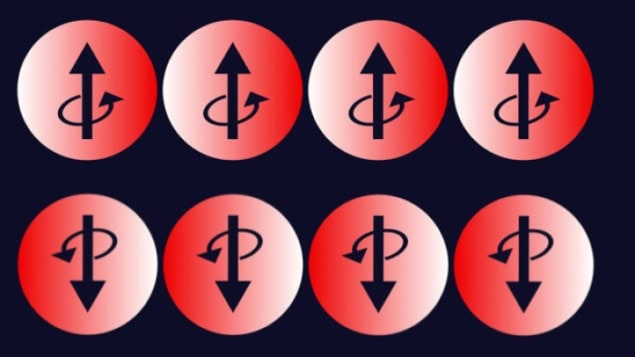
Two Bose-Einstein condensates (BECs) of magnetic atoms have been created just 50 nm apart from each other – giving physicists the first opportunity to study atomic interactions on this length scale. The work by physicists in the US could lead to studies of several interesting collective phenomena in quantum physics, and could even be useful in quantum computing.
First created in 1995, BECs have become invaluable tools for studying quantum physics. A BEC is a macroscopic entity comprising thousands of atoms that are described by a single quantum wavefunction. They are created by cooling a trapped cloud of bosonic atoms to a temperature so low that a large fraction of the atoms are in the lowest energy (ground) state of the system.
BECs should be ideal for studying the quantum physics of exotic, strongly interacting systems. However, to prolong the lifetime of a BEC, physicists need to keep it isolated from the outside world to prevent decoherence. This need for isolation makes it difficult to manoeuvre BECs close enough together for the interactions to be studied.
Pancake layers
In the new work, researchers at Massachusetts Institute of Technology in the group of Wolfgang Ketterle (who shared the 2001 Nobel Prize for Physics for cresting BECs) tackled this problem by creating a double-layer BEC of dysprosium atoms, with the two layers just 50 nm apart. To achieve this, the researchers had to keep two pancake-like condensate layers a constant distance apart using lasers with wavelengths more than ten times their separation. This would have been almost impossible using separate optical traps.
Instead, the researchers utilized the fact that dysprosium has a very large spin magnetic moment. They lifted the degeneracy of two electronic spin states using an applied magnetic field. Atoms with opposite spins coupled to light with slightly different frequencies and opposite polarizations. The researchers sent light at both frequencies down the same optical fibre onto the same mirror. Both beams formed standing waves in the cavity. “If the frequency of these two standing waves is slightly different, then at the position where we load this bilayer array, these two standing waves are going to slightly walk off,” says Li Du, who is lead author on the paper describing the research. “Therefore by tuning the frequency difference we’re able to tune the interlayer separation,” he adds.
As both beams utilize the same optical fibre and the same mirror, they are robust to physical disturbance of these components. “Our scheme guarantees that you have two standing waves that can shake a little – or maybe a lot – but the shaking is a common mode, so the difference between the two layers is always fixed,” says Du.
Ringing atoms
The researchers heated one of the layers by about 2 μK and showed how the heat flowed across the vacuum gap to the other layer through the magnetic coupling of the atomic dipoles. Next they induced oscillations in the position of one layer and showed how these affected the position of the other layer: “We hit one layer with a hammer and we see that the other [layer] also starts to ring,” says Du.
The researchers now hope to use the platform to study how atoms closer together than one photon wavelength interact with light. “If the separation is much smaller than the wavelength of light, then the light can no longer tell [the atoms] apart,” says Du. “That potentially allows us to study a special effect called super-radiance.”
Beyond this, the researchers would like to investigate the work’s potential in quantum computing: “We would really like to implement a magnetic quantum gate purely driven by the magnetic dipole-dipole interaction,” he says. The same platform could also be used with BECs of molecules, which would open up the study of electric dipole–dipole interactions. Indeed, in late 2023, researchers at Columbia University in the US published a preprint that describes how they created a BEC of dipolar molecules. This preprint has yet to be peer reviewed.
Twisted graphene
Experimental atomic physicist Cheng Chin of the University of Chicago in Illinois, who last year collaborated with researchers at Shanxi University in China to produce a double layer of rubidium atoms to model twisted bilayer graphene, says that Ketterle and colleagues’ research is “very, very interesting”.
‘Alice rings’ spotted in a Bose–Einstein condensate
He adds, “This is the first time we’re able to prepare cold atom systems in two layers with such a small spacing…To control such a 2D system is hard but necessary in order to induce the interaction that’s required in two planes. It’s a very smart choice of atom because dysprosium has a very large dipole-dipole interaction. At a conventional spacing of half a micron, you wouldn’t be able to see any kind of coupling between the two layers, but 50 nm is just enough to show that the atoms in the two planes can really talk to each other.”
He suggests follow-up work from both teams’ research could focus on simulating new phases of matter and simulating emergent ones such as superconducting bilayer graphene.
The research is described in Science.
- SEO Powered Content & PR Distribution. Get Amplified Today.
- PlatoData.Network Vertical Generative Ai. Empower Yourself. Access Here.
- PlatoAiStream. Web3 Intelligence. Knowledge Amplified. Access Here.
- PlatoESG. Carbon, CleanTech, Energy, Environment, Solar, Waste Management. Access Here.
- PlatoHealth. Biotech and Clinical Trials Intelligence. Access Here.
- Source: https://physicsworld.com/a/bilayer-of-ultracold-atoms-has-just-a-50-nm-gap/
- :has
- :is
- :where
- $UP
- 160
- 1995
- 2001
- 2023
- 2D
- 4
- 50
- a
- Able
- About
- Achieve
- across
- Adds
- affected
- alice
- allows
- almost
- also
- always
- an
- and
- any
- apart
- applied
- ARE
- Array
- AS
- At
- atom
- author
- BE
- BEC
- because
- become
- been
- between
- both
- but
- by
- called
- CAN
- chicago
- China
- choice
- Close
- closer
- Cloud
- cold
- collaborated
- Collective
- Columbia
- Common
- components
- comprising
- computing
- constant
- control
- conventional
- could
- coupled
- courtesy
- created
- Creating
- described
- describes
- describing
- difference
- different
- difficult
- distance
- double
- down
- driven
- each
- effect
- Electric
- Electronic
- energy
- enough
- entity
- Even
- Exotic
- fact
- field
- First
- first time
- fixed
- Focus
- For
- formed
- fraction
- Frequency
- from
- gap
- gate
- Giving
- going
- Graphene
- Ground
- Group
- guarantees
- had
- Half
- hammer
- Hard
- Have
- he
- Hit
- hope
- How
- However
- HTTPS
- ideal
- illinois
- implement
- impossible
- in
- indeed
- information
- Institute
- interact
- interacting
- interaction
- interactions
- interesting
- invaluable
- investigate
- isolated
- isolation
- issue
- IT
- jpg
- just
- Keep
- Kind
- large
- lasers
- Last
- Last Year
- Late
- layer
- layers
- lead
- Length
- lifetime
- Lifted
- light
- like
- little
- load
- longer
- Lot
- Low
- lowest
- Magnetic field
- MAKES
- massachusetts
- Massachusetts Institute of technology
- Matter
- max-width
- maybe
- micron
- mirror
- MIT
- Mode
- model
- moment
- more
- much
- Nature
- necessary
- Need
- New
- next
- no
- nobel
- nobel prize
- now
- of
- off
- on
- ONE
- ones
- onto
- open
- Opportunity
- opposite
- optical
- or
- order
- Other
- outside
- Paper
- peer
- phases
- Phases of Matter
- physical
- physicist
- Physics
- Physics World
- Planes
- platform
- plato
- Plato Data Intelligence
- PlatoData
- position
- potential
- potentially
- Prepare
- prevent
- prize
- Problem
- produce
- published
- purely
- Quantum
- quantum computing
- quantum gate
- quantum physics
- really
- required
- research
- researchers
- reviewed
- Ring
- robust
- same
- says
- Scale
- scheme
- see
- sent
- separate
- several
- shared
- should
- show
- showed
- single
- slightly
- slightly different
- small
- smaller
- smart
- So
- special
- Spin
- spins
- standing
- starts
- State
- States
- strongly
- studied
- studies
- Study
- Studying
- such
- Suggests
- system
- Systems
- Talk
- Technology
- tell
- ten
- than
- that
- The
- their
- then
- These
- they
- this
- thousands
- Through
- thumbnail
- time
- times
- to
- together
- tools
- trapped
- traps
- trouble
- true
- tune
- tuning
- twisted
- two
- university
- University of Chicago
- us
- use
- used
- useful
- using
- utilize
- utilized
- Vacuum
- very
- walk
- wavelengths
- waves
- we
- which
- WHO
- with
- Work
- world
- would
- year
- yet
- you
- zephyrnet