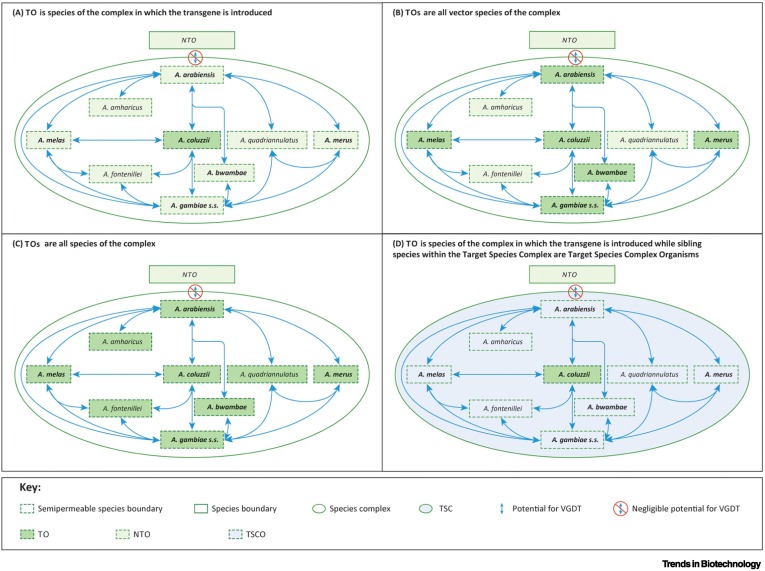
Site-specific selfish genes as tools for the control and genetic engineering of natural populations.
Proc. Biol. Sci. 2003; 270: 921-928
Gene drive to reduce malaria transmission in sub-Saharan Africa.
J. Responsible Innov. 2018; 5: S66-S80
Symbionts and gene drive: two strategies to combat vector-borne disease.
Trends Genet. 2022; 38: 708-723
Gene Drives for Malaria Control and Elimination in Africa.
2018
Evaluation of Genetically Modified Mosquitoes for the Control of Vector-Borne Diseases.
WHO, 2020
Gene Drives on the Horizon: Advancing Science, Navigating Uncertainty, and Aligning Research with Public Values.
National Academies Press, 2016
A general risk-based adaptive management scheme incorporating the Bayesian Network Relative Risk Model with the South River, Virginia, as case study.
Integr. Environ. Assess. Manag. 2017; 13: 115-126
Pathway to deployment of gene drive mosquitoes as a potential biocontrol tool for elimination of malaria in Sub-Saharan Africa: recommendations of a Scientific Working Group.
Am. J. Trop. Med. Hyg. 2018; 98: 1-49
Adequacy and sufficiency evaluation of existing EFSA guidelines for the molecular characterisation, environmental risk assessment and post-market environmental monitoring of genetically modified insects containing engineered gene drives.
EFSA J. 2020; 18e06297
Toward the definition of efficacy and safety criteria for advancing gene drive-modified mosquitoes to field testing.
Vector Borne Zoonotic Dis. 2020; 20: 237-251
Guidance Framework for Testing Genetically Modified Mosquitoes.
2nd edn. WHO, 2021
Predicting the spread and persistence of genetically modified dominant sterile male mosquitoes.
Parasit. Vectors. 2021; 14: 480
Recommendations for environmental risk assessment of gene drive applications for malaria vector control.
Malar. J. 2022; 21: 152
The value of existing regulatory frameworks for the environmental risk assessment of agricultural pest control using gene drives.
Environ. Sci. Policy. 2020; 108: 19-36
Gene drive-modified organisms: developing practical risk assessment guidance.
Trends Biotechnol. 2021; 39: 853-856
Potential use of gene drive modified insects against disease vectors, agricultural pests and invasive species poses new challenges for risk assessment.
Crit. Rev. Biotechnol. 2021; : 1-17
Risk management recommendations for environmental releases of gene drive modified insects.
Biotechnol. Adv. 2021; 107807
Anopheles gambiae, a complex of species.
Bull. World Health Organ. 1964; 31: 625-634
Semipermeable species boundaries between Anopheles gambiae and Anopheles arabiensis: evidence from multilocus DNA sequence variation.
Proc. Natl. Acad. Sci. U. S. A. 2003; 100: 10818-10823
Radiation with reticulation marks the origin of a major malaria vector.
Proc. Natl. Acad. Sci. U. S. A. 2020; 117: 31583-31590
Highlights on anopheles nili and anopheles moucheti, malaria vectors in Africa.
in: Anopheles Mosquitoes – New insights into Malaria Vectors. IntechOpen, 2013
Reticulate speciation and barriers to introgression in the Anopheles gambiae species complex.
Genome Biol. Evol. 2015; 7: 3116-3131
Evidence for mitochondrial introgression between Anopheles bwambae and Anopheles gambiae.
Insect Mol. Biol. 2000; 9: 203-210
A CRISPR-Cas9 gene drive targeting doublesex causes complete population suppression in caged Anopheles gambiae mosquitoes.
Nat. Biotechnol. 2018; 36: 1062-1066
Gene-drive suppression of mosquito populations in large cages as a bridge between lab and field.
Nat. Commun. 2021; 12: 4589
The dominant Anopheles vectors of human malaria in Africa, Europe and the Middle East: occurrence data, distribution maps and bionomic precis.
Parasit. Vectors. 2010; 3: 117
Secondary malaria vectors of Sub-Saharan Africa: threat to malaria elimination on the continent?.
in: Rodriguez-Morales A.J. Current Topics in Malaria. IntechOpen, 2016
Gene drive: evolved and synthetic.
ACS Chem. Biol. 2018; 13: 343-346
Chromosomal evidence for natural interspecific hybridization by mosquitoes of the Anopheles gambiae complex.
Nature. 1971; 231: 184-185
Living at the edge: biogeographic patterns of habitat segregation conform to speciation by niche expansion in anopheles gambiae.
BMC Ecol. 2009; 9: 16
Dissecting functional components of reproductive isolation among closely related sympatric species of the anopheles gambiae complex.
Evol. Appl. 2017; 10: 1102-1120
Seasonal malaria vector and transmission dynamics in western Burkina Faso.
Malar. J. 2019; 18: 113
Updated list of anopheles species (Diptera: Culicidae) by country in the Afrotropical Region and associated islands.
Zootaxa. 2020; 4747 ()
A geo-coded inventory of anophelines in the Afrotropical Region south of the Sahara: 1898-2016.
Wellcome Open Res. 2017; 2: 57
Modelling the suppression of a malaria vector using a CRISPR-Cas9 gene drive to reduce female fertility.
BMC Biol. 2020; 18: 98
Systematic identification of plausible pathways to potential harm via problem formulation for investigational releases of a population suppression gene drive to control the human malaria vector Anopheles gambiae in West Africa.
Malar. J. 2021; 20
Speciation in Anopheles gambiae – the distribution of genetic polymorphism and patterns of reproductive isolation among natural populations.
in: Manguin S. Anopheles Mosquitoes – New Insights into Malaria Vectors. IntechOpen, 2013
Genetic diversity of the African malaria vector Anopheles gambiae.
Nature. 2017; 552: 96-100
Targeting male mosquito mating behaviour for malaria control.
Parasit. Vectors. 2015; 8: 347
Does extreme asymmetric dominance promote hybridization between Anopheles coluzzii and Anopheles gambiae s.s. in seasonal malaria mosquito communities of West Africa?.
Parasit. Vectors. 2015; 8: 586
Selection of non-target Lepidoptera species to test Bt maize effects in the laboratory: which species and how to breed them?.
BioRisk. 2020; 15: 45-65
When the average hides the risk of Bt-corn pollen on non-target Lepidoptera: Application to Aglais io in Catalonia.
Ecotoxicol. Environ. Saf. 2021; 207111215
Transfer of Cry1Ac and Cry2Ab proteins from genetically engineered Bt cotton to herbivores and predators.
Insect Sci. 2017; 25: 823-832
Bt rice in China – focusing the nontarget risk assessment.
Plant Biotechnol. J. 2017; 15: 1340-1345
Successful suppression of a field mosquito population by sustained release of engineered male mosquitoes.
Nat. Biotechnol. 2012; 30: 828-830
Suppression of a field population of Aedes aegypti in Brazil by sustained release of transgenic male mosquitoes.
PLoS Negl. Trop. Dis. 2015; 9e0003864
Short-term suppression of Aedes aegypti using genetic control does not facilitate Aedes albopictus.
Pest Manag. Sci. 2016; 72: 618-628
Anopheles bwambae sp.n., a malaria vector in the Semliki Valley, Uganda, and its relationships with other sibling species of the An. gambiae complex (Diptera: Culicidae).
Syst. Entomol. 1985; 10: 501-522
Colonisation and mass rearing: learning from others.
Malar. J. 2009; 8: S4
Fitness characteristics of the malaria vector Anopheles funestus during an attempted laboratory colonization.
Malar. J. 2021; 20: 148
Genetic pest management and the background genetics of release strains.
Philos. Trans. R. Soc. Lond. Ser. B Biol. Sci. 2021; 37620190805
Electrophoretic and DNA identification of Anopheles bwambae and A. gambiae (Diptera: Culicidae) in western Uganda.
Bull. Entomol. Res. 2007; 89: 111-117
Adaptive introgression between Anopheles sibling species eliminates a major genomic island but not reproductive isolation.
Nat. Commun. 2014; 5: 4248
Adaptive introgression in an African malaria mosquito coincident with the increased usage of insecticide-treated bed nets.
Proc. Natl. Acad. Sci. U. S. A. 2015; 112: 815-820
Adaptive potential of hybridization among malaria vectors: introgression at the immune locus TEP1 between Anopheles coluzzii and A. gambiae in ‘Far-West’ Africa.
PLoS One. 2015; 10e0127804
The ecology of Bactrocera tryoni (Diptera: Tephritidae): what do we know to assist pest management?.
Ann. Appl. Biol. 2011; 158: 26-54
Genomic architecture and introgression shape a butterfly radiation.
Science. 2019; 366: 594-599
Ancient hybridization fuels rapid cichlid fish adaptive radiations.
Nat. Commun. 2017; 8: 14363
Candidate-species delimitation in Desmognathus salamanders reveals gene flow across lineage boundaries, confounding phylogenetic estimation and clarifying hybrid zones.
Ecol. Evol. 2022; 12e8574
Species delimitation with gene flow: a methodological comparison and population genomics approach to elucidate cryptic species boundaries in Malaysian Torrent Frogs.
Mol. Ecol. 2017; 26: 5435-5450
The challenge of species delimitation at the extremes: diversification without morphological change in philippine sun skinks.
Evolution. 2013; 67: 3556-3572
Evolution of Darwin’s finches and their beaks revealed by genome sequencing.
Nature. 2015; 518: 371-375
Adaptive introgression of anticoagulant rodent poison resistance by hybridization between old world mice.
Curr. Biol. 2011; 21: 1296-1301
Population genomics reveals speciation and introgression between Brown Norway Rats and their sibling species.
Mol. Biol. Evol. 2017; 34: 2214-2228
Consequences of hybridization in mammals: a systematic review.
Genes (Basel). 2021; 13: 50
Opinion: standardizing the definition of gene drive.
Proc. Natl. Acad. Sci. U. S. A. 2020; 117: 30864-30867
Means and ends of effective global risk assessments for genetic pest management.
BMC Proc. 2018; 12: 13
World Malaria Report.
WHO, 2021
Gene drives to fight malaria: current state and future directions.
Pathog. Glob. Health. 2018; 111: 412-423
A CRISPR-Cas9 gene drive system targeting female reproduction in the malaria mosquito vector Anopheles gambiae.
Nat. Biotechnol. 2016; 34: 78-83
Mosquito genomics. Extensive introgression in a malaria vector species complex revealed by phylogenomics.
Science. 2015; 3471258524
A global map of dominant malaria vectors.
Parasit. Vectors. 2012; 5: 69
Unexpected diversity of Anopheles species in Eastern Zambia: implications for evaluating vector behavior and interventions using molecular tools.
Sci. Rep. 2015; 5: 17952
Anopheles coluzzii and Anopheles amharicus, new members of the Anopheles gambiae complex.
Zootaxa. 2013; 3619: 246-274
A new species in the major malaria vector complex sheds light on reticulated species evolution.
Sci. Rep. 2019; 9: 14753
A cryptic subgroup of Anopheles gambiae is highly susceptible to human malaria parasites.
Science. 2011; 331: 596-598
Evolution of GOUNDRY, a cryptic subgroup of Anopheles gambiae s.l., and its impact on susceptibility to Plasmodium infection.
Mol. Ecol. 2016; 25: 1494-1510
A population genomic unveiling of a new cryptic mosquito taxon within the malaria-transmitting Anopheles gambiae complex.
Mol. Ecol. 2021; 30: 775-790
Anopheles gambiae complex.
Nature. 1962; 196: 907
The five mating-types in the Anopheles gambiae complex.
Riv. Malariol. 1964; 43: 167-183
The Anopheles gambiae complex.
in: Wright J.W. Pal R. Genetics of Insect Vectors of Disease. Elsevier, 1967: 211-250
Chromosomal differentiation and adaptation to human environments in the Anopheles gambiae complex.
Trans. R. Soc. Trop. Med. Hyg. 1979; 73: 483-497
Detection of hybrids in natural populations of the Anopheles gambiae complex by the rDNA-based, PCR method.
Ann. Trop. Med. Parasitol. 1997; 91: 963-965
Molecular evidence of incipient speciation within Anopheles gambiae s.s. in West Africa.
Insect Mol. Biol. 2001; 10: 9-18
Spatiotemporal dynamics of gene flow and hybrid fitness between the M and S forms of the malaria mosquito, Anopheles gambiae.
Proc. Natl. Acad. Sci. U. S. A. 2013; 110: 19854-19859
Contemporary gene flow between wild An. gambiae s.s. and An. arabiensis.
Parasit. Vectors. 2014; 7: 345
Mosquito genomics. Highly evolvable malaria vectors: the genomes of 16 Anopheles mosquitoes.
Science. 2015; 3471258522
Guidance on the environmental risk assessment of genetically modified animals.
EFSA J. 2013; 11: 3200
- SEO Powered Content & PR Distribution. Get Amplified Today.
- Platoblockchain. Web3 Metaverse Intelligence. Knowledge Amplified. Access Here.
- Source: https://www.cell.com/trends/biotechnology/fulltext/S0167-7799(22)00167-6?rss=yes
- 1
- a
- across
- africa
- African
- against
- agency
- Agricultural
- among
- and
- animals
- Application
- applications
- approach
- architecture
- assessment
- assessments
- assist
- associated
- attempted
- authors
- average
- background
- barriers
- Bayesian
- between
- boundaries
- Brazil
- BREED
- BRIDGE
- BT
- case
- case study
- causes
- challenge
- challenges
- change
- characteristics
- China
- closely
- combat
- Communities
- comparison
- complete
- complex
- components
- continent
- control
- country
- criteria
- Current
- Current state
- data
- defining
- deployment
- developing
- Development
- Disease
- diseases
- distribution
- diversification
- Diversity
- dna
- Dominance
- dominant
- drive
- during
- dynamics
- East
- eastern
- Edge
- Effective
- effects
- eliminates
- ends
- Engineering
- environmental
- environments
- Europe
- evaluation
- evidence
- evolution
- evolved
- existing
- expansion
- extensive
- extreme
- extremes
- facilitate
- female
- field
- fight
- Fish
- fitness
- flow
- focusing
- forms
- Framework
- frameworks
- frogs
- from
- functional
- future
- General
- genetic engineering
- Genetics
- genome
- genomics
- Global
- Group
- guidelines
- Health
- highly
- horizon
- How
- How To
- HTTPS
- human
- Hybrid
- Identification
- Impact
- implications
- in
- incorporating
- increased
- infection
- insights
- inventory
- island
- Islands
- isolation
- Know
- lab
- laboratory
- large
- learning
- light
- List
- major
- malaria
- management
- map
- Maps
- Mass
- medicine
- Members
- method
- mice
- Middle
- Middle East
- model
- modified
- MOL
- molecular
- monitoring
- mosquito
- Natural
- navigating
- Nets
- network
- New
- Norway
- Old
- ONE
- open
- organization
- Origin
- Other
- Others
- panel
- Partnership
- patterns
- PCR
- persistence
- Philippine
- plato
- Plato Data Intelligence
- PlatoData
- plausible
- poison
- policy
- Pollen
- population
- populations
- poses
- potential
- Practical
- predators
- Problem
- PROC
- promote
- Proteins
- public
- Radiation
- rapid
- recommendations
- reduce
- region
- regulatory
- related
- Relationships
- release
- Releases
- report
- reproduction
- research
- Resistance
- responsible
- Revealed
- Reveals
- review
- Rice
- Risk
- risk assessment
- River
- Safety
- scheme
- SCI
- Science
- SCIENCES
- Sequence
- Shape
- South
- spread
- standardizing
- State
- Strains
- strategies
- Study
- SUB-SAHARAN
- Sun
- suppression
- susceptible
- synthetic
- system
- Target
- targeting
- test
- Testing
- The
- their
- threat
- to
- tool
- tools
- Topics
- Torrent
- Uganda
- Uncertainty
- union
- unveiling
- Usage
- use
- Valley
- value
- Values
- via
- virginia
- W
- West
- west africa
- Western
- What
- which
- Wild
- within
- without
- working
- Working Group
- world
- zephyrnet
- zones