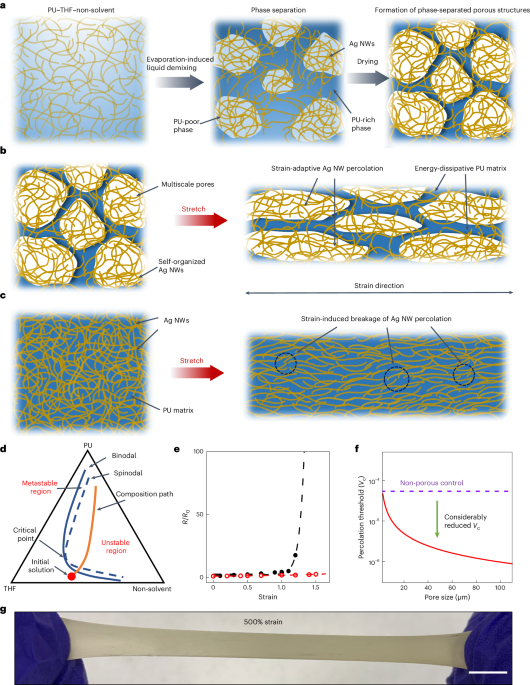
Jung, D. et al. Highly conductive and elastic nanomembrane for skin electronics. Science 373, 1022–1026 (2021).
Choi, S. et al. Highly conductive, stretchable and biocompatible Ag–Au core–sheath nanowire composite for wearable and implantable bioelectronics. Nat. Nanotechnol. 13, 1048–1056 (2018).
Lee, W. et al. Universal assembly of liquid metal particles in polymers enables elastic printed circuit board. Science 378, 637–641 (2022).
Matsuhisa, N. et al. Printable elastic conductors by in situ formation of silver nanoparticles from silver flakes. Nat. Mater. 16, 834–840 (2017).
Veerapandian, S. et al. Hydrogen-doped viscoplastic liquid metal microparticles for stretchable printed metal lines. Nat. Mater. 20, 533–540 (2021).
Hui, Y. et al. Three-dimensional printing of soft hydrogel electronics. Nat. Electron. 5, 893–903 (2022).
Jiang, Z. et al. A 1.3-micrometre-thick elastic conductor for seamless on-skin and implantable sensors. Nat. Electron. 5, 784–793 (2022).
Zhao, Y. et al. A self-healing electrically conductive organogel composite. Nat. Electron. 6, 206–215 (2023).
Jiang, Z. et al. Highly stretchable metallic nanowire networks reinforced by the underlying randomly distributed elastic polymer nanofibers via interfacial adhesion improvement. Adv. Mater. 31, 1903446 (2019).
Luo, Y. et al. Technology roadmap for flexible sensors. ACS Nano 17, 5211–5295 (2023).
Wang, C., He, T., Zhou, H., Zhang, Z. & Lee, C. Artificial intelligence enhanced sensors—enabling technologies to next-generation healthcare and biomedical platform. Bioelectron. Med. 9, 17 (2023).
Choi, S., Han, S. I., Kim, D., Hyeon, T. & Kim, D.-H. High-performance stretchable conductive nanocomposites: materials, processes, and device applications. Chem. Soc. Rev. 48, 1566–1595 (2019).
Sim, K., Rao, Z., Ershad, F. & Yu, C. Rubbery electronics fully made of stretchable elastomeric electronic materials. Adv. Mater. 32, 1902417 (2020).
Gao, W. et al. Fully integrated wearable sensor arrays for multiplexed in situ perspiration analysis. Nature 529, 509–514 (2016).
Yang, Y. & Gao, W. Wearable and flexible electronics for continuous molecular monitoring. Chem. Soc. Rev. 48, 1465–1491 (2019).
Kim, J. J. et al. Skin electronics: next‐generation device platform for virtual and augmented reality. Adv. Funct. Mater. 31, 2009602 (2021).
Yamagishi, K., Zhou, W., Ching, T., Huang, S. Y. & Hashimoto, M. Ultra‐deformable and tissue‐adhesive liquid metal antennas with high wireless powering efficiency. Adv. Mater. 33, 2008062 (2021).
Zhuang, Q. et al. Wafer-patterned, permeable, and stretchable liquid metal microelectrodes for implantable bioelectronics with chronic biocompatibility. Sci. Adv. 9, eadg8602 (2023).
Jung, D. et al. Adaptive self‐organization of nanomaterials enables strain‐insensitive resistance of stretchable metallic nanocomposites. Adv. Mater. 34, 2200980 (2022).
Wang, T., Liu, Q., Liu, H., Xu, B. & Xu, H. Printable and highly stretchable viscoelastic conductors with kinematically reconstructed conductive pathways. Adv. Mater. 34, 2202418 (2022).
Kim, S. H. et al. An ultrastretchable and self-healable nanocomposite conductor enabled by autonomously percolative electrical pathways. ACS Nano 13, 6531–6539 (2019).
Sun, B. et al. Gas‐permeable, multifunctional on‐skin electronics based on laser‐induced porous graphene and sugar‐templated elastomer sponges. Adv. Mater. 30, 1804327 (2018).
Yi, H. et al. Constructing high-performance 3D porous self-standing electrodes with various morphologies and shapes by a flexible phase separation-derived method. J. Mater. Chem. A 7, 22550–22558 (2019).
Wang, Y., Yokota, T. & Someya, T. Electrospun nanofiber-based soft electronics. NPG Asia Mater. 13, 22 (2021).
Zhu, D., Handschuh-Wang, S. & Zhou, X. Recent progress in fabrication and application of polydimethylsiloxane sponges. J. Mater. Chem. A 5, 16467–16497 (2017).
Yao, S. et al. Ultrasoft porous 3D conductive dry electrodes for electrophysiological sensing and myoelectric control. Adv. Mater. Technol. 7, 2101637 (2022).
Zhou, W. et al. Gas-permeable, ultrathin, stretchable epidermal electronics with porous electrodes. ACS Nano 14, 5798–5805 (2020).
Wang, F. et al. Progress report on phase separation in polymer solutions. Adv. Mater. 31, 1806733 (2019).
Hołda, A. K. & Vankelecom, I. F. Understanding and guiding the phase inversion process for synthesis of solvent resistant nanofiltration membranes. J. Appl. Polym. Sci. 132, 42130 (2015).
Mandal, J. et al. Hierarchically porous polymer coatings for highly efficient passive daytime radiative cooling. Science 362, 315–319 (2018).
Zhu, H.-W. et al. Printable elastic silver nanowire-based conductor for washable electronic textiles. Nano Res. 13, 2879–2884 (2020).
Lee, H. J., Jung, B., Kang, Y. S. & Lee, H. Phase separation of polymer casting solution by nonsolvent vapor. J. Membr. Sci. 245, 103–112 (2004).
Sun, X., Sun, G. & Wang, X. Morphology modeling for polymer monolith obtained by non-solvent-induced phase separation. Polymer 108, 432–441 (2017).
Binks, B. & Lumsdon, S. Pickering emulsions stabilized by monodisperse latex particles: effects of particle size. Langmuir 17, 4540–4547 (2001).
Hu, N., Karube, Y., Yan, C., Masuda, Z. & Fukunaga, H. Tunneling effect in a polymer/carbon nanotube nanocomposite strain sensor. Acta Mater. 56, 2929–2936 (2008).
Amjadi, M., Pichitpajongkit, A., Lee, S., Ryu, S. & Park, I. Highly stretchable and sensitive strain sensor based on silver nanowire–elastomer nanocomposite. ACS Nano 8, 5154–5163 (2014).
Li, J. et al. Correlations between percolation threshold, dispersion state, and aspect ratio of carbon nanotubes. Adv. Funct. Mater. 17, 3207–3215 (2007).
Taherian, R. Development of an equation to model electrical conductivity of polymer-based carbon nanocomposites. ECS J. Solid State Sci. Technol. 3, M26–M38 (2014).
Liu, Y. et al. Capillary-force-induced cold welding in silver-nanowire-based flexible transparent electrodes. Nano Lett. 17, 1090–1096 (2017).
Zheng, X. et al. Ultralight, ultrastiff mechanical metamaterials. Science 344, 1373–1377 (2014).
Liu, S., Rao, Y., Jang, H., Tan, P. & Lu, N. Strategies for body-conformable electronics. Matter 5, 1104–1136 (2022).
Kim, Y. et al. Stretchable nanoparticle conductors with self-organized conductive pathways. Nature 500, 59–63 (2013).
Boland, C. S. et al. Sensitive electromechanical sensors using viscoelastic graphene–polymer nanocomposites. Science 354, 1257–1260 (2016).
Sun, H., Han, Z. & Willenbacher, N. Ultrastretchable conductive elastomers with a low percolation threshold for printed soft electronics. ACS Appl. Mater. Interfaces 11, 38092–38102 (2019).
Liu, Y., Ji, X. & Liang, J. Rupture stress of liquid metal nanoparticles and their applications in stretchable conductors and dielectrics. Npj Flex. Electron. 5, 11 (2021).
Ma, Z. et al. Permeable superelastic liquid-metal fibre mat enables biocompatible and monolithic stretchable electronics. Nat. Mater. 20, 859–868 (2021).
Chung, H. U. et al. Binodal, wireless epidermal electronic systems with in-sensor analytics for neonatal intensive care. Science 363, eaau0780 (2019).
Ouyang, W. et al. A wireless and battery-less implant for multimodal closed-loop neuromodulation in small animals. Nat. Biomed. Eng. 7, 1252–1269 (2023).
Jiang, Y. et al. Wireless, closed-loop, smart bandage with integrated sensors and stimulators for advanced wound care and accelerated healing. Nat. Biotechnol. 41, 652–662 (2023).
Zhang, Y. et al. High precision epidermal radio frequency antenna via nanofiber network for wireless stretchable multifunction electronics. Nat. Commun. 11, 5629 (2020).
Liu, T.-L. et al. Battery-free, tuning circuit–inspired wireless sensor systems for detection of multiple biomarkers in bodily fluids. Sci. Adv. 8, eabo7049 (2022).
Park, S. I. et al. Soft, stretchable, fully implantable miniaturized optoelectronic systems for wireless optogenetics. Nat. Biotechnol. 33, 1280–1286 (2015).
Someya, T., Bao, Z. & Malliaras, G. G. The rise of plastic bioelectronics. Nature 540, 379–385 (2016).
- SEO Powered Content & PR Distribution. Get Amplified Today.
- PlatoData.Network Vertical Generative Ai. Empower Yourself. Access Here.
- PlatoAiStream. Web3 Intelligence. Knowledge Amplified. Access Here.
- PlatoESG. Carbon, CleanTech, Energy, Environment, Solar, Waste Management. Access Here.
- PlatoHealth. Biotech and Clinical Trials Intelligence. Access Here.
- Source: https://www.nature.com/articles/s41565-024-01658-6
- ][p
- 02
- 08
- 1
- 10
- 11
- 12
- 13
- 14
- 15%
- 16
- 17
- 19
- 2%
- 20
- 2001
- 2007
- 2008
- 2013
- 2014
- 2015
- 2016
- 2017
- 2018
- 2019
- 2020
- 2021
- 2022
- 2023
- 21
- 22
- 23
- 24
- 25
- 26
- 27
- 28
- 29
- 30
- 31
- 32
- 33
- 34
- 35%
- 36
- 39
- 3d
- 4
- 40
- 41
- 42
- 45
- 46
- 48
- 49
- 5
- 50
- 51
- 52
- 6
- 7
- 8
- 9
- a
- accelerated
- adaptive
- advanced
- AL
- an
- analysis
- analytics
- and
- animals
- antenna
- Application
- applications
- article
- artificial
- artificial intelligence
- asia
- aspect
- Assembly
- augmented
- Augmented Reality
- autonomously
- b
- based
- between
- biomarkers
- biomedical
- board
- by
- carbon
- carbon nanotubes
- care
- casting
- central
- circuit
- click
- cold
- conductivity
- conductor
- constructing
- continuous
- control
- correlations
- Detection
- Development
- device
- Dispersion
- distributed
- dry
- E&T
- effect
- effects
- efficiency
- efficient
- Electronic
- Electronics
- enabled
- enables
- enhanced
- equation
- Ether (ETH)
- flexible
- For
- formation
- Frequency
- from
- fully
- GAO
- Graphene
- guiding
- he
- healing
- healthcare
- High
- high-performance
- highly
- http
- HTTPS
- huang
- i
- improvement
- in
- integrated
- Intelligence
- intensive
- inversion
- Kim
- Lee
- lines
- LINK
- Liquid
- Low
- made
- materials
- matt
- mechanical
- metal
- metamaterials
- method
- model
- modeling
- molecular
- monitoring
- Monolithic
- multiple
- Nanomaterials
- nanotechnology
- Nature
- network
- networks
- next-generation
- NIH
- obtained
- of
- on
- Park
- particle
- passive
- pathways
- phase
- plastic
- platform
- plato
- Plato Data Intelligence
- PlatoData
- polymer
- Polymers
- Powering
- Precision
- printing
- process
- processes
- Progress
- R
- Radio
- ratio
- Reality
- recent
- reference
- reinforced
- report
- Resistance
- resistant
- Rise
- roadmap
- s
- Scholar
- SCI
- seamless
- sensitive
- sensors
- shapes
- Silver
- Size
- Skin
- small
- smart
- Soft
- solid
- solution
- Solutions
- State
- strategies
- stress
- Sun
- synthesis
- Systems
- T
- Technologies
- Technology
- textiles
- The
- their
- three-dimensional
- threshold
- to
- transparent
- tuning
- underlying
- understanding
- Universal
- using
- various
- via
- Virtual
- W
- wang
- washable
- wearable
- wireless
- with
- X
- zephyrnet
- zhang