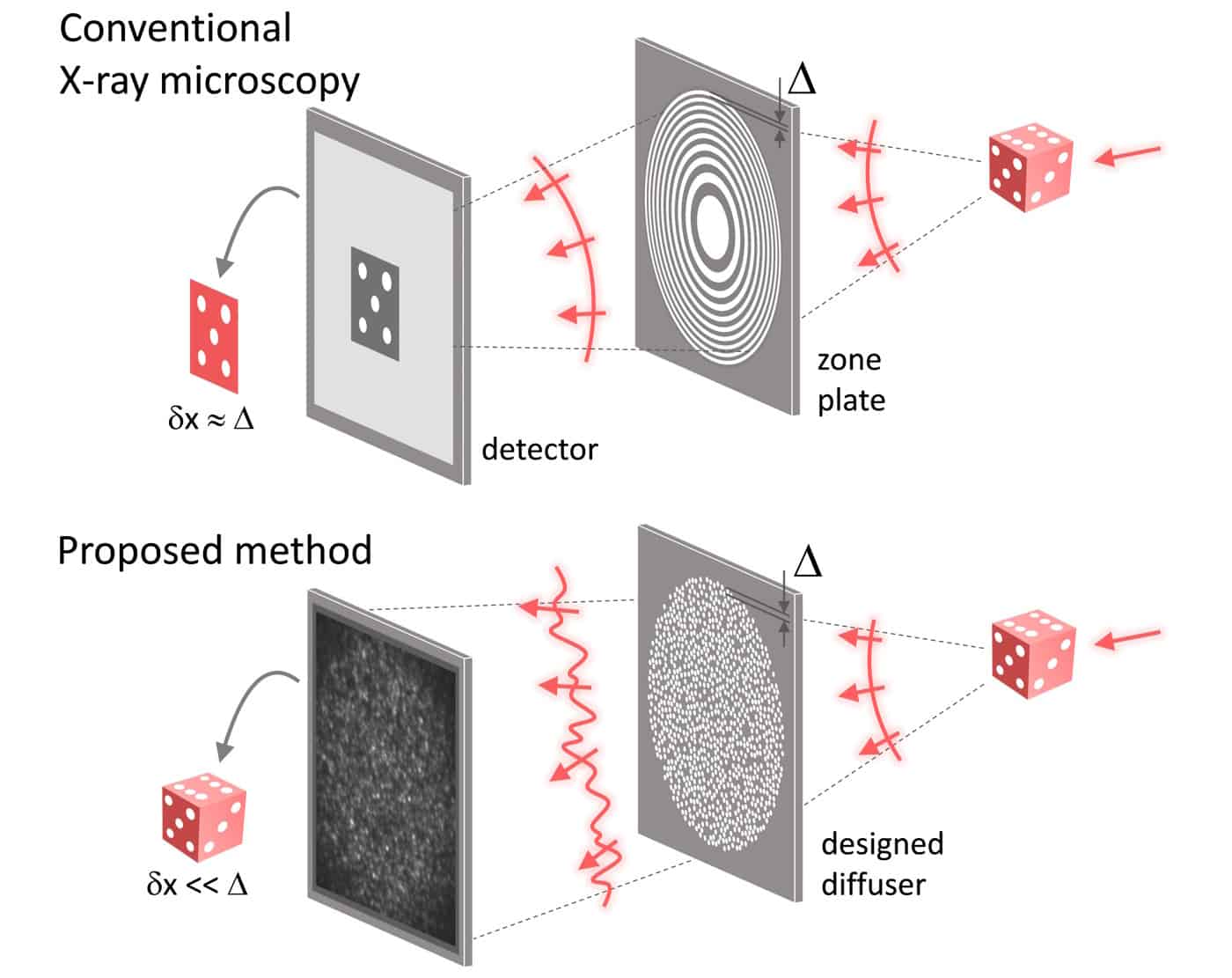
A new X-ray microscopy technique could make it possible to image objects in finer detail, overcoming the spatial resolution limits of today’s X-ray imaging technologies. Developed by researchers in Korea, the technique relies on an X-ray diffuser made from a metal film speckled with tiny holes, and its single-shot spatial resolution of 14 nm is already smaller than the size of the holes. According to the researchers, the resolution could be improved still further by using next-generation X-ray light sources and high-performance X-ray detectors.
Because X-rays can penetrate most objects, they are a popular tool for characterizing materials as well as imaging bones and other biological structures. The resolution of X-ray imaging is, however, limited by the difficulty of constructing optics for very short wavelengths of light.
Unlike optical microscopy, which uses refractive lenses to focus and manipulate visible light, X-ray microscopy typically relies on circular gratings known as zone plates. The quality of the nanostructures in these plates determines the spatial resolution of the image, but manufacturing such structures to the desired tolerances is challenging, and once built, they are prone to collapse because of their thin, comb-like nature. The result is that the resolution of X-ray microscopy has never approached its theoretical (diffraction) limit and is instead restricted by practicalities.
A new X-ray “lens”
Physicists KyeoReh Lee and YongKeun Park of the Korea Advanced Institute of Science and Technology (KAIST) have now overcome this limitation by cleverly exploiting the random nature of diffraction. Working with colleagues at the Pohang Accelerator Laboratory (PAL), they constructed their X-ray diffuser by punching numerous holes in a thin tungsten film. When this diffuser is placed behind the sample being imaged, it diffracts the light, generating a pattern of speckles. At a first glance, this speckle pattern may appear unrelated to the incident light, but Lee explains that a high-resolution sample image can nevertheless be retrieved from it by exploiting the mathematical properties of random diffraction.
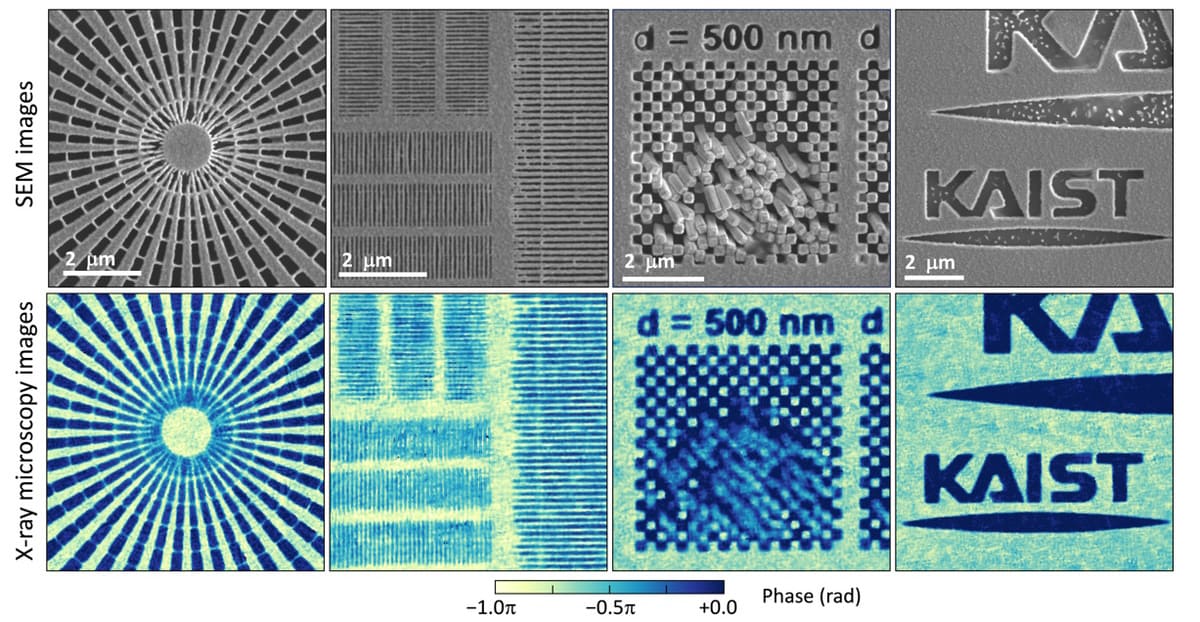
The team first demonstrated this randomness-based imaging technique using visible light in 2016, and the contrast with traditional X-ray microscopy methods is striking, Lee says. “In conventional zone-plate-based X-ray microscopy, the finer outermost zone width is used to collect higher-angle diffracted photons that contain higher-resolution features,” he tells Physics World. “On the contrary, in this work, we do not collect the photons at all. Instead, we measure the phase of high-angle diffracted photons by exploiting the pseudorandomness, and reconstruct the high-resolution image computationally.”
Lee and colleagues say their new technique could substantially improve the resolution of X-ray microscopy, especially at very high energies (the so-called hard X-ray regime) where making high-resolution zone plates is more difficult. Possible applications could include non-invasive observations of the fine structures present in nanoscale samples of battery materials, ceramics, semiconductors and more.
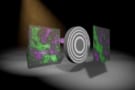
New technique produces colour X-ray images quickly and efficiently
While the technique shows promise, the researchers acknowledge that its current resolution of 14 nm is “not very impressive” comparted to alternatives. Lee, however, argues that using next-generation X-ray light sources such as diffraction-limited storage rings along with high-performance X-ray detectors could pave the way for much higher resolutions. The ultimate goal, he adds, is to reach sub-nanometre image resolution using X-rays.
“In principle, increasing the size of the diffuser and detector in the demonstrated setup could potentially attain the desired resolution, but for non-crystalline samples, it may be difficult to obtain sufficient diffracted photons for detection,” Lee concludes.
The present work is detailed in Light: Science and Applications.
- SEO Powered Content & PR Distribution. Get Amplified Today.
- PlatoAiStream. Web3 Data Intelligence. Knowledge Amplified. Access Here.
- Minting the Future w Adryenn Ashley. Access Here.
- Buy and Sell Shares in PRE-IPO Companies with PREIPO®. Access Here.
- Source: https://physicsworld.com/a/pseudorandomness-enhances-x-ray-microscopy/
- :has
- :is
- :not
- :where
- 14
- 2016
- a
- AC
- accelerator
- According
- acknowledge
- Adds
- advanced
- All
- along
- already
- alternatives
- an
- and
- appear
- applications
- ARE
- Argues
- artistic
- AS
- At
- battery
- BE
- because
- behind
- being
- biomedical
- Bottom
- built
- but
- by
- CAN
- challenging
- click
- Collapse
- colleagues
- collect
- constructing
- contrary
- contrast
- conventional
- Corresponding
- could
- created
- Current
- demonstrated
- designed
- desired
- detail
- detailed
- Detection
- determines
- developed
- difficult
- Difficulty
- do
- due
- DX
- Enhances
- especially
- Explains
- Features
- Film
- fine
- First
- Focus
- For
- from
- further
- generating
- Glance
- goal
- Green
- Hard
- Have
- he
- High
- high-performance
- high-resolution
- higher
- Hole
- Holes
- How
- However
- HTTPS
- image
- images
- Imaging
- improve
- improved
- in
- incident
- include
- increasing
- information
- instead
- Institute
- issue
- IT
- ITS
- jpg
- known
- korea
- laboratory
- Lee
- left
- Lens
- lenses
- light
- LIMIT
- limitation
- Limited
- limits
- made
- make
- Making
- manufacturing
- map
- Match
- materials
- mathematical
- max-width
- May..
- measure
- metal
- method
- methods
- Microscope
- Microscopy
- more
- most
- much
- Nature
- never
- Nevertheless
- New
- next-generation
- now
- numerous
- objects
- obtain
- of
- on
- once
- open
- optical
- optics
- Other
- Overcome
- Pattern
- pave
- phase
- Photons
- Physics
- Physics World
- plato
- Plato Data Intelligence
- PlatoData
- Popular
- possible
- potentially
- present
- principle
- promise
- properties
- proposed
- quality
- quickly
- random
- reach
- regime
- representation
- researchers
- Resolution
- restricted
- result
- say
- says
- scanning
- Science
- Science and Technology
- SEM
- Semiconductors
- setup
- Short
- Shows
- Size
- smaller
- Sources
- Spatial
- Still
- storage
- such
- sufficient
- team
- Technologies
- Technology
- tells
- than
- that
- The
- their
- theoretical
- These
- they
- this
- thumbnail
- to
- today’s
- tool
- top
- traditional
- true
- two
- typically
- ultimate
- used
- using
- very
- visible
- wavelengths
- Way..
- we
- WELL
- when
- which
- with
- Work
- working
- world
- x-ray
- zephyrnet