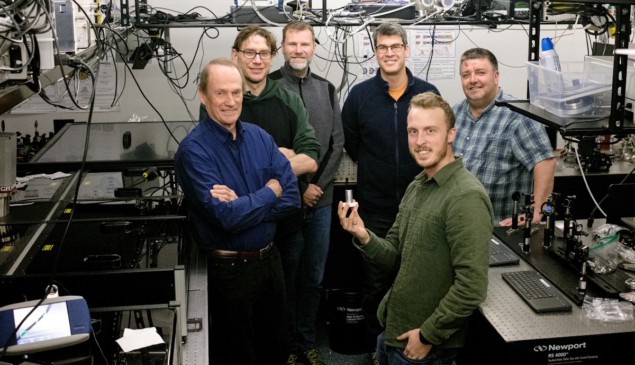
During his time as a postdoctoral researcher at the Institut National de la Recherche Scientifique (INRS) in Canada, Simon Vallières was approached by a colleague who had made a puzzling observation. The colleague was creating a plasma in air using a newly upgraded laser at INRS’s Advanced Laser Light Source (ALLS) Laboratory when they noticed that the readings on their Geiger counter were higher than expected.
“He was focusing the laser, which was running at 100 Hz, in air and putting a Geiger counter close to the focal spot. Even three metres away from the focal spot, his Geiger counter was clicking,” says Vallières, now a research associate at INRS. “That’s quite a far range for X-rays or electrons to travel. I said, maybe we should measure [the dose being delivered] with well-calibrated dosimeters.”
Medical physicists from the McGill University Health Centre measured the radiation dose from the experimental setup with three independently calibrated radiation detectors. Doses were measured over eight orders of magnitude at distances up to 6 m from the laser focus, as well as for different angles at fixed distances. They used absolute dose calibrations to confirm the data.
The laser had been upgraded from a µJ- to a mJ-class high average power laser. And now, with the laser tightly focused and tuned to an opportunistic set of parameters to create a plasma in air, an electron beam reaching up to 1.4 MeV at a dose rate of 0.15 Gy/s was produced. The researchers’ finding pushes the boundaries of our knowledge about high-power laser pulses, radiation safety and maybe even FLASH radiotherapy, an emerging cancer treatment technique.
Operating with optimal parameters
“Our models ruled out other acceleration mechanisms that could have played a role. We narrowed it down to one explanation: this was acceleration from the laser electric field, known as ponderomotive acceleration,” Vallières says.
The researchers were operating the laser in a regime that ionized air molecules and then harnessed the laser’s electric field to accelerate the resulting electrons above 1 MeV.
“If you tell laser physicists that you can focus a laser in air and produce 1 MeV electrons, no-one will believe it. That’s because the more energy you put into laser pulses, during the focusing period, you will accumulate nonlinear effects which will destroy the shape of the beam, and you will saturate in intensity. But it turns out that we were very lucky,” Vallières says. “The wavelength, pulse duration and focal length all played a role.”
Vallières explains that the researchers were operating the laser in the mid-infrared part of the electromagnetic spectrum. By using a longer wavelength than most high average power lasers (1.8 µm instead of around 800 nm), nonlinear aberrations were reduced. This wavelength is also ideal for creating a plasma at near-critical density, contributing to a high dose per pulse.
The researchers also used a short laser pulse (12 fs). This reduced the nonlinear refractive index – a parameter related to the electrons that oscillate in air molecules and the rotation of air molecules themselves – by approximately 75%, which also limited nonlinear effects.
With tight focusing (a short focal length), the researchers again drastically reduced nonlinear effects. Ultimately, the laser reached a high enough intensity (peak intensities up to 1019 W/cm2) to kick out electrons at up to 1.4 MeV.
FLASH, radiation safety applications
Infinite Potential Laboratories LP has provided funding for the researchers to push R&D forward and develop related technologies, and at least one patent is pending.
One application of interest is the FLASH effect. Compared with conventional radiation therapy techniques, FLASH radiotherapy can be used to rapidly deliver high doses of radiation to better protect the healthy tissue around a tumour. Instantaneous dose rates of the electron bunches produced by the researchers’ laser-based system are orders of magnitude higher than medical linear accelerators, even those driven in FLASH mode.
“No study has been able to explain the mechanism behind the FLASH effect yet,” says Vallières. “We hope we can develop a cell or mice radiation platform to study the radiobiology of FLASH.”
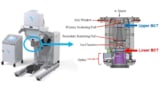
High-energy physics devices adapted for electron FLASH dosimetry
Lessons in radiation safety are also a high priority for Vallières. Today’s high-average-power lasers now produce laser beams with intensities as high as the largest lasers of the early 2000s, and at much higher repetition rates – leading to high dose rates. The researchers hope that this work improves field-level knowledge and leads to radiation safety regulations.
“The electron energies we observed allow them to travel more than three metres in air. We unravelled a big radiation hazard,” Vallières says. “I have presented this work at conferences, people are shocked… It’s true, I mean, who aligns a focusing parabola with a Geiger counter? We did this because it’s something we’ve done in the past. I think [this work] is just going to open peoples’ eyes a bit more and they’re going to be more careful when they create a plasma in air. We hope to change the laser safety regulation through this work.”
The research is described in Laser & Photonics Reviews.
- SEO Powered Content & PR Distribution. Get Amplified Today.
- PlatoData.Network Vertical Generative Ai. Empower Yourself. Access Here.
- PlatoAiStream. Web3 Intelligence. Knowledge Amplified. Access Here.
- PlatoESG. Carbon, CleanTech, Energy, Environment, Solar, Waste Management. Access Here.
- PlatoHealth. Biotech and Clinical Trials Intelligence. Access Here.
- Source: https://physicsworld.com/a/ultrafast-laser-based-electron-beam-could-help-explore-radiobiology-of-the-flash-effect/
- :has
- :is
- $UP
- 1
- 100
- 12
- 15%
- 160
- 2000s
- 8
- 90
- a
- Able
- About
- above
- Absolute
- accelerate
- acceleration
- accelerators
- Accumulate
- adapted
- again
- AIR
- Aligns
- All
- allow
- also
- an
- and
- Application
- approximately
- ARE
- around
- AS
- Associate
- At
- average
- away
- BE
- Beam
- because
- been
- behind
- being
- believe
- Better
- Big
- Bit
- boundaries
- but
- by
- CAN
- Canada
- Cancer
- cancer treatment
- careful
- cell
- change
- Close
- colleague
- compared
- conferences
- Confirm
- contributing
- conventional
- could
- Counter
- create
- Creating
- data
- de
- deliver
- delivered
- density
- described
- destroy
- develop
- Devices
- DID
- different
- done
- dose
- doses
- down
- drastically
- driven
- duration
- during
- Early
- effect
- effects
- eight
- Electric
- electrons
- emerging
- energy
- enough
- Ether (ETH)
- Even
- expected
- experimental
- Explain
- Explains
- explanation
- explore
- Eyes
- far
- field
- finding
- fixed
- Flash
- focal
- Focus
- focused
- focusing
- For
- Forward
- from
- FS
- funding
- going
- had
- Have
- Health
- healthy
- help
- High
- higher
- his
- hope
- HTTPS
- i
- ideal
- image
- improves
- in
- independently
- index
- information
- instead
- interest
- into
- issue
- IT
- jpg
- just
- kick
- knowledge
- known
- la
- laboratories
- largest
- laser
- lasers
- leading
- Leads
- least
- left
- Length
- light
- Limited
- longer
- LP
- made
- max-width
- maybe
- mean
- measure
- measured
- mechanism
- mechanisms
- medical
- MEV
- mice
- Mode
- models
- more
- most
- much
- National
- newly
- now
- observation
- observed
- of
- on
- ONE
- open
- operating
- optimal
- or
- orders
- Other
- our
- out
- over
- parameter
- parameters
- part
- past
- patent
- Peak
- pending
- People
- per
- period
- Physics
- Physics World
- Plasma
- platform
- plato
- Plato Data Intelligence
- PlatoData
- played
- potential
- power
- presented
- priority
- produce
- Produced
- protect
- provided
- pulse
- Push
- pushes
- put
- Putting
- quite
- R&D
- Radiation
- Radiotherapy
- range
- rapidly
- Rate
- Rates
- reached
- reaching
- Reduced
- regime
- Regulation
- regulations
- related
- research
- researcher
- researchers
- resulting
- right
- Role
- ruled
- running
- Safety
- safety regulations
- Said
- says
- set
- setup
- Shape
- Short
- should
- Simon
- something
- Source
- Spectrum
- Spot
- Steve
- Study
- system
- team
- technique
- techniques
- Technologies
- tell
- than
- that
- The
- their
- Them
- themselves
- then
- therapy
- they
- Think
- this
- those
- three
- Through
- thumbnail
- tightly
- time
- tissue
- to
- today’s
- travel
- treatment
- true
- tuned
- turns
- Ultimately
- university
- upgraded
- used
- using
- very
- was
- we
- WELL
- were
- when
- which
- WHO
- will
- with
- Work
- world
- yet
- you
- zephyrnet