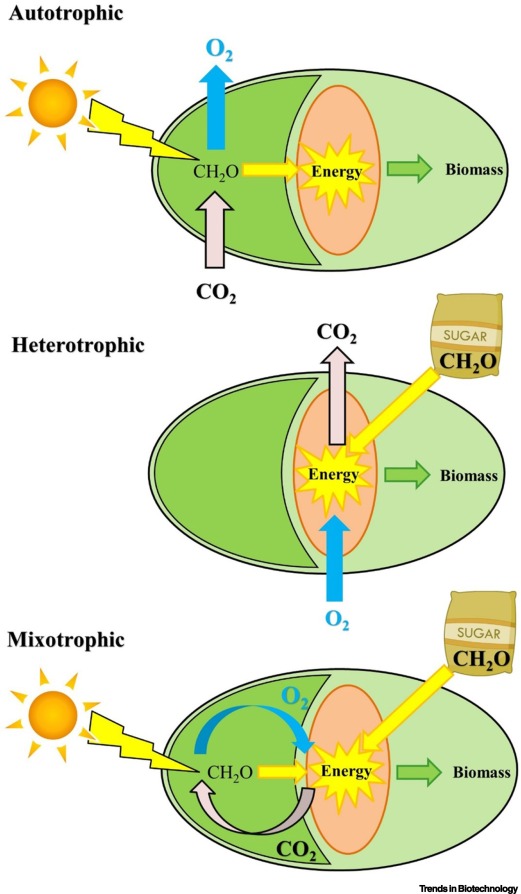
Photobioreactors: Design and performance with respect to energy input light.
Adv. Biochem. Eng. Biotechnol. 1998; 59: 123-152
Algae burgers for a hungry world? The rise and fall of Chlorella cuisine.
Technol. Cult. 1997; 38: 606-634
My sixty years in applied algology.
J. Appl. Phycol. 2003; 15: 99-106
Commercial microalgal cultivation systems.
in: Hallmann A. Rampelotto P. Grand Challenges in Algae Biotechnology. Springer, 2019: 3-34
Microalgae based production of single-cell protein.
Curr. Opin. Biotechnol. 2022; 75102705
Towards microalgal triglycerides in the commodity markets.
Biotechnol. Biofuels. 2017; 10: 188
Doubling of microalgae productivity by oxygen balanced mixotrophy.
ACS Sustain. Chem. Eng. 2020; 8: 6065-6074
Mixotrophic cultivation of Galdieria sulphuraria for C-phycocyanin and protein production.
Algal Res. 2022; 61102603
Photovoltaic-driven microbial protein production can use land and sunlight more efficiently than conventional crops.
Proc. Natl. Acad. Sci. U. S. A. 2021; 118e2015025118
Life cycle and functional genomics of the unicellular red alga Galdieria for elucidating algal and plant evolution and industrial use.
Proc. Natl. Acad. Sci. U. S. A. 2022; 119e2210665119
Autotrophic and mixotrophic biomass production of the acidophilic Galdieria sulphuraria ACUF 64.
Algal Res. 2021; 60102513
Evolutionary pathways and enigmatic algae: Cyanidium caldarium (Rhodophyta) and related cells.
Springer, 1994
Heterotrophic high cell-density fed-batch cultures of the phycocyanin-producing red alga Galdieria sulphuraria.
Biotechnol. Bioeng. 2005; 90: 77-84
Bioprospecting and characterization of temperature tolerant microalgae from Bonaire.
Algal Res. 2020; 50102008
Growth parameter estimation and model simulation for three industrially relevant microalgae: Picochlorum, Nannochloropsis, and Neochloris.
Biotechnol. Bioeng. 2022; 119: 1416-1425
Development of a high-productivity, halophilic, thermotolerant microalga Picochlorum renovo.
Commun. Biol. 2019; 2: 388
High-light selection produces a fast-growing Picochlorum celeri.
Algal Res. 2018; 36: 17-28
Solar cultivation of microalgae in a desert environment for the development of techno-functional feed ingredients for aquaculture in Qatar.
Sci. Total Environ. 2022; 835155538
Global phosphorus shortage will be aggravated by soil erosion.
Nat. Commun. 2020; 11: 4546
Nitrogen management and the future of food: Lessons from the management of energy and carbon.
Proc. Natl. Acad. Sci. U. S. A. 1999; 96: 6001-6008
Report from the Commission to the Council and the European Parliament on the implementation of Council Directive 91/676/EEC concerning the protection of waters against pollution caused by nitrates from agricultural sources based on Member State reports.
2021
Green revolution: Impacts, limits, and the path ahead.
Proc. Natl. Acad. Sci. U. S. A. 2012; 109: 12302-12308
Nutrient enrichment and eutrophication in Europe’s seas moving towards a healthy marine environment.
2019
An outlook on microalgal biofuels.
Science. 2010; 329: 796-799
Comprehensive review of water management and wastewater treatment in food processing industries in the framework of water-food-environment nexus.
Compr. Rev. Food Sci. Food Saf. 2021; 20: 4779-4815
Microalgae-based wastewater treatment: Mechanisms, challenges, recent advances, and future prospects.
Environ. Sci. Ecotechnol. 2022; 13100205
The lipid biochemistry of eukaryotic algae.
Prog. Lipid Res. 2019; 74: 31-68
The impact of nitrogen starvation on the dynamics of triacylglycerol accumulation in nine microalgae strains.
Bioresour. Technol. 2012; 124: 217-226
Impact of sustainable feeds on omega-3 long-chain fatty acid levels in farmed Atlantic salmon, 2006-2015.
Sci. Rep. 2016; 6: 21892
Lipid production from Nannochloropsis.
Mar. Drugs. 2016; 14https://doi.org/10.3390/md14040061
Omega-3 biotechnology: Errors and omissions.
Biotechnol. Adv. 2012; 30: 1746-1747
Omega-3 long-chain polyunsaturated fatty acids, EPA and DHA: Bridging the gap between supply and demand.
Nutrients. 2019; 11: 89
Towards sustainable sources for omega-3 fatty acids production.
Curr. Opin. Biotechnol. 2014; 26: 14-18
Microalgal biofactories: A promising approach towards sustainable omega-3 fatty acid production.
Microb. Cell Factories. 2012; 11: 1
A nutritionally-enhanced oil from transgenic Camelina sativa effectively replaces fish oil as a source of eicosapentaenoic acid for fish.
Sci. Rep. 2015; 5: 8104
Tailoring seed oil composition in the real world: Optimising omega-3 long chain polyunsaturated fatty acid accumulation in transgenic Camelina sativa.
Sci. Rep. 2017; 7: 1-12
Production of omega-3 eicosapentaenoic acid by metabolic engineering of Yarrowia lipolytica.
Nat. Biotechnol. 2013; 31: 734-740
Sustainable source of omega-3 eicosapentaenoic acid from metabolically engineered Yarrowia lipolytica: From fundamental research to commercial production.
Appl. Microbiol. Biotechnol. 2015; 99: 1599-1610
Can we approach theoretical lipid yields in microalgae?.
Trends Biotechnol. 2018; 36: 265-276
Microalgae for oil: Strain selection, induction of lipid synthesis and outdoor mass cultivation in a low-cost photobioreactor.
Biotechnol. Bioeng. 2009; 102: 100-112
Selecting microalgae with high lipid productivity and photosynthetic activity under nitrogen starvation.
J. Appl. Phycol. 2015; 27: 1425-1431
Batch and semi-continuous microalgal TAG production in lab-scale and outdoor photobioreactors.
J. Appl. Phycol. 2016; 28: 3167-3177
Unlocking nature’s treasure-chest: screening for oleaginous algae.
Sci. Rep. 2015; 5: 9844
Lipid production in Nannochloropsis gaditana is doubled by decreasing expression of a single transcriptional regulator.
Nat. Biotechnol. 2017; 35: 647-652
Genetic engineering of microalgae for enhanced lipid production.
Biotechnology Advances. vol. 52. Elsevier Inc., 2021https://doi.org/10.1016/j.biotechadv.2021.107836
High-throughput insertional mutagenesis reveals novel targets for enhancing lipid accumulation in Nannochloropsis oceanica.
Metab. Eng. 2021; 66: 239-258
Stress-induced neutral lipid biosynthesis in microalgae – Molecular, cellular and physiological insights.
Biochim. Biophys. Acta Mol. Cell Biol. Lipids. 2016; 1861: 1269-1281
Microalgae, old sustainable food and fashion nutraceuticals.
Microb. Biotechnol. 2017; 10: 1017-1024
Evaluation of the potential of 9 Nannochloropsis strains for biodiesel production.
Bioresour. Technol. 2014; 167: 503-509
Year-round sustainable biomass production potential of Nannochloris sp. in outdoor raceway pond enabled through strategic photobiological screening.
Photosynth. Res. 2022; https://doi.org/10.1007/s11120-022-00984-x
Genome, functional gene annotation, and nuclear transformation of the heterokont oleaginous alga Nannochloropsis oceanica CCMP1779.
PLoS Genet. 2012; 8https://doi.org/10.1371/journal.pgen.1003064
Nannochloropsis genomes reveal evolution of microalgal oleaginous traits.
PLoS Genet. 2014; 10https://doi.org/10.1371/journal.pgen.1004094
Choreography of transcriptomes and lipidomes of Nannochloropsis reveals the mechanisms of oil synthesis in microalgae.
Plant Cell. 2014; 26: 1645-1665
Genome editing of model oleaginous microalgae Nannochloropsis spp. by CRISPR/Cas9.
Plant J. 2016; 88https://doi.org/10.1111/tpj.13307
A type 2 diacylglycerol acyltransferase accelerates the triacylglycerol biosynthesis in heterokont oleaginous microalga Nannochloropsis oceanica.
J. Biotechnol. 2016; 229: 65-71
Metabolic profiles of Nannochloropsis oceanica IMET1 under nitrogen-deficiency stress.
Bioresour. Technol. 2013; 130: 731-738
Accumulation of medium chain fatty acids in Nannochloropsis oceanica by heterologous expression of Cuphea palustris thioesterase FatB1.
Algal Res. 2022; 64https://doi.org/10.1016/j.algal.2022.102665
The nucleolus as a genomic safe harbor for strong gene expression in Nannochloropsis oceanica.
Mol. Plant. 2022; 15: 340-353
Comprehensive genome engineering toolbox for microalgae Nannochloropsis oceanica based on CRISPR-Cas systems.
ACS Synth. Biol. 2021; 10: 3369-3378
CRISPR–Cas ribonucleoprotein mediated homology-directed repair for efficient targeted genome editing in microalgae Nannochloropsis oceanica IMET1.
Biotechnol. Biofuels. 2019; 12: 66
Microalgae of the genus Nannochloropsis: Chemical composition and functional implications for human nutrition.
Journal of Functional Foods. 68. Elsevier Ltd, 2020https://doi.org/10.1016/j.jff.2020.103919
Picochlorum celeri as a model system for robust outdoor algal growth in seawater.
Sci. Rep. 2021; 11https://doi.org/10.1038/s41598-021-91106-5
Development of the high-productivity marine microalga, Picochlorum renovo, as a photosynthetic protein secretion platform.
Algal Res. 2021; 54https://doi.org/10.1016/j.algal.2021.102197
Role of an ancient light-harvesting protein of PSI in light absorption and photoprotection.
Nat. Commun. 2021; 12https://doi.org/10.1038/s41467-021-20967-1
High-efficiency nuclear transformation of the microalgae Nannochloropsis oceanica using Tn5 transposome for the generation of altered lipid accumulation phenotypes.
Biotechnol. Biofuels. 2019; 12https://doi.org/10.1186/s13068-019-1475-y
Development and characterization of a Nannochloropsis mutant with simultaneously enhanced growth and lipid production.
Biotechnol. Biofuels. 2020; 13https://doi.org/10.1186/s13068-020-01681-4
The NanDeSyn database for Nannochloropsis systems and synthetic biology.
Plant J. 2020; 104: 1736-1745
Draft genome sequence and genetic transformation of the oleaginous alga Nannochloropis gaditana.
Nat. Commun. 2012; 3: 610-686
RNAi-based targeted gene knockdown in the model oleaginous microalgae Nannochloropsis oceanica.
Plant J. 2017; 89: 1236-1250
A CRISPR/dCas9-based transcription activated system developed in marine microalga Nannochloropsis oceanica.
Aquaculture. 2022; 546https://doi.org/10.1016/j.aquaculture.2021.737064
Development of a constitutive and an auto-inducible high-yield expression system for recombinant protein production in the microalga Nannochloropsis oceanica.
Appl. Microbiol. Biotechnol. 2020; 104: 8747-8760
Modern subunit vaccines: Development, components, and research opportunities.
ChemMedChem. 2013; 8: 360-376
Recent advances in oral vaccine development: Yeast-derived β-glucan particles.
Hum. Vaccin. Immunother. 2014; 10: 1309-1318
Novel antigen delivery systems.
World J. Virol. 2015; 4: 156
Chloroplast-derived vaccines against human diseases: Achievements, challenges and scopes.
Plant Biotechnol. J. 2011; 9: 527-539
Plant-produced vaccines: promise and reality.
Drug Discov. Today. 2009; 14: 16-24
Robust expression of a bioactive mammalian protein in Chlamydomonas chloroplast.
Plant Biotechnol. J. 2007; 5: 402-412
Algae-based oral recombinant vaccines.
Frontiers in Microbiology. vol. 5, no. FEB. Frontiers Research Foundation, 2014https://doi.org/10.3389/fmicb.2014.00060
Evaluation of microalgae as immunostimulants and recombinant vaccines for diseases prevention and control in aquaculture.
Frontiers in Bioengineering and Biotechnology. vol. 8. Frontiers Media S.A., 2020https://doi.org/10.3389/fbioe.2020.590431
Enhanced chloroplast transgene expression in a nuclear mutant of Chlamydomonas.
Plant Biotechnol. J. 2011; 9: 565-574
Preparation of transgenic Dunaliella salina for immunization against white spot syndrome virus in crayfish.
Arch. Virol. 2014; 159: 519-525
Oral administration of Anabaena-expressed VP28 for both drug and food against white spot syndrome virus in shrimp.
J. Appl. Phycol. 2016; 28: 1001-1009
Effect of trans-vp28 gene Synechocystis sp. PCC6803 on growth and immunity of Litopenaeus vannamei and defense against white spot syndrome virus (WSSV).
Aquaculture. 2019; 512https://doi.org/10.1016/j.aquaculture.2019.734306
Heat-stable oral alga-based vaccine protects mice from Staphylococcus aureus infection.
J. Biotechnol. 2010; 145: 273-280
Development of spirulina for the manufacture and oral delivery of protein therapeutics.
Nat. Biotechnol. 2022; 40: 956-964
Recent developments in bioprocessing of recombinant proteins: Expression hosts and process development.
Frontiers in Bioengineering and Biotechnology. vol. 7. Frontiers Media S.A., 2019https://doi.org/10.3389/fbioe.2019.00420
Critical analysis of the commercial potential of plants for the production of recombinant proteins.
Front. Plant Sci. 2019; 10https://doi.org/10.3389/fpls.2019.00720
Viral contamination in biologic manufacture and implications for emerging therapies.
Nature Biotechnology. vol. 38, no. 5. Nature Research, 2020: 563-572
Stable integration and functional expression of flounder growth hormone gene in transformed microalga, Chlorella ellipsoidea.
Mar. Biotechnol. (NY). 2002; 4https://doi.org/10.1007/s1012601-0070-x
Expression and assembly of a fully active antibody in algae.
Proc. Natl. Acad. Sci. U. S. A. 2003; 100: 438-442
Production of therapeutic proteins in algae, analysis of expression of seven human proteins in the chloroplast of Chlamydomonas reinhardtii.
Plant Biotechnol. J. 2010; 8: 719-733
Algae as protein factories: Expression of a human antibody and the respective antigen in the diatom Phaeodactylum tricornutum.
PLoS One. 2011; 6https://doi.org/10.1371/journal.pone.0028424
Production of recombinant and therapeutic proteins in microalgae.
Current Opinion in Biotechnology. vol. 78. Elsevier Ltd, 2022https://doi.org/10.1016/j.copbio.2022.102784
Transient glyco-engineering to produce recombinant IgA1 with defined N-and O-glycans in plants.
Front. Plant Sci. 2016; 7https://doi.org/10.3389/fpls.2016.00018
Production of recombinant proteins by microbes and higher organisms.
Biotechnol. Adv. 2009; 27: 297-306
Recombinant proteins for industrial versus pharmaceutical purposes: A review of process and pricing.
Processes. 2019; 7: 476
A brief reminder of systems of production and chromatography-based recovery of recombinant protein biopharmaceuticals.
BioMed Research International. vol. 2019. Hindawi Limited, 2019https://doi.org/10.1155/2019/4216060
Protein glycoengineering: An approach for improving protein properties.
Frontiers in Chemistry. vol. 8. Frontiers Media S.A., 2020https://doi.org/10.3389/fchem.2020.00622
The potential for microalgae as bioreactors to produce pharmaceuticals.
Int. J. Mol. Sci. 2016; 17: 962
Biotechnological insights on the expression and production of antimicrobial peptides in plants.
Molecules. vol. 26, no. 13. MDPI AG, 2021https://doi.org/10.3390/molecules26134032
Cultivation of microalgae on artificial light comes at a cost.
Algal Res. 2013; 2: 333-340
Oxygen balanced mixotrophy in microalgae.
Wageningen University, Wageningen2021
Towards industrial products from microalgae.
Energy Environ. Sci. 2016; 9: 3036-3043
Techno-economic assessment of microalgae production, harvesting and drying for food, feed, cosmetics, and agriculture.
Sci. Total Environ. 2022; 837https://doi.org/10.1016/j.scitotenv.2022.155742
High variability in nutritional value and safety of commercially available Chlorella and Spirulina biomass indicates the need for smart production strategies.
Bioresour. Technol. 2019; 275: 247-257
Chemical composition of Spirulina and eukaryotic algae food products marketed in Spain.
J. Appl. Phycol. 1993; 5: 425-435
Single cell protein-state-of-the-art, industrial landscape and patents 2001-2016.
Frontiers in Microbiology. vol. 8, no. OCT. Frontiers Media S.A., 2017https://doi.org/10.3389/fmicb.2017.02009
An integrated review of factors influencing the performance of photovoltaic panels’.
Renewable and Sustainable Energy Reviews. vol. 80. Elsevier Ltd, 2017: 1499-1511
Fishmeal and fish oil production and trade flows in the EU.
- SEO Powered Content & PR Distribution. Get Amplified Today.
- Platoblockchain. Web3 Metaverse Intelligence. Knowledge Amplified. Access Here.
- Source: https://www.cell.com/trends/biotechnology/fulltext/S0167-7799(22)00345-6?rss=yes
- 1
- 2014
- 2016
- 2017
- 2019
- 2020
- 2021
- 2022
- 7
- 9
- a
- accelerates
- accumulation
- achievements
- active
- activity
- administration
- advances
- against
- agency
- Agricultural
- agriculture
- ahead
- analysis
- Ancient
- and
- applied
- approach
- artificial
- Assembly
- assessment
- authors
- available
- based
- between
- biology
- biomass
- biotechnology
- bridging
- carbon
- caused
- chain
- challenges
- chemical
- chemistry
- commercial
- commercially
- commission
- commodity
- components
- control
- conventional
- Cost
- Council
- crops
- cult
- cultivation
- Database
- Defense
- delivery
- Demand
- Design
- developed
- Development
- developments
- diseases
- doubled
- drug
- Drugs
- dynamics
- effectively
- efficient
- efficiently
- emerging
- Emerging therapies
- enabled
- energy
- Engineering
- enhanced
- enhancing
- Environment
- EPA
- Errors
- EU
- European
- European Parliament
- Europes
- evolution
- factories
- factors
- Fall
- Fashion
- Fish
- Flows
- food
- foods
- Forward
- Framework
- from
- fully
- functional
- fundamental
- future
- Future of Food
- gap
- generation
- genome
- genomics
- Growth
- Harvesting
- healthy
- High
- higher
- hopes
- HTTPS
- human
- Hungry
- immunity
- Impact
- Impacts
- implementation
- implications
- improving
- in
- indicates
- industrial
- industries
- infection
- influencing
- input
- insights
- integrated
- integration
- International
- Land
- landscape
- Lessons
- levels
- light
- limits
- List
- Long
- management
- Marine
- Market
- Markets
- Mass
- Media
- medium
- member
- mice
- microbiology
- model
- MOL
- molecular
- more
- moving
- Need
- Neutral
- nexus
- novel
- nuclear
- nutrition
- NY
- observatory
- Oct
- Oil
- Old
- ONE
- Opinion
- opportunities
- Outdoor
- Outlook
- Oxygen
- parameter
- parliament
- Patents
- path
- performance
- Pharmaceutical
- pharmaceuticals
- plants
- platform
- plato
- Plato Data Intelligence
- PlatoData
- Pollution
- POND
- potential
- Prevention
- pricing
- process
- processing
- produce
- Production
- productivity
- Products
- Profiles
- promise
- promising
- properties
- prospects
- protection
- Protein
- Proteins
- purposes
- Qatar
- real
- real world
- Reality
- recent
- recovery
- Red
- regulator
- related
- relevant
- repair
- Reports
- research
- respective
- reveal
- Reveals
- review
- Reviews
- Revolution
- Rise
- robust
- safe
- Safety
- SCI
- screening
- seed
- selection
- Sequence
- seven
- shortage
- simulation
- simultaneously
- single
- smart
- Source
- Sources
- Spain
- Spot
- State
- Strains
- Strategic
- strategies
- stress
- strong
- sunlight
- supply
- Supply and Demand
- sustainable
- Sustainable Energy
- synthetic
- system
- Systems
- TAG
- targeted
- targets
- The
- The Future
- theoretical
- Therapeutic
- therapeutics
- three
- Through
- to
- today
- Toolbox
- Total
- towards
- trade
- Transformation
- transformed
- treatment
- under
- use
- Vaccine
- vaccines
- value
- Versus
- virus
- W
- Water
- white
- will
- world
- X
- years
- yields
- zephyrnet