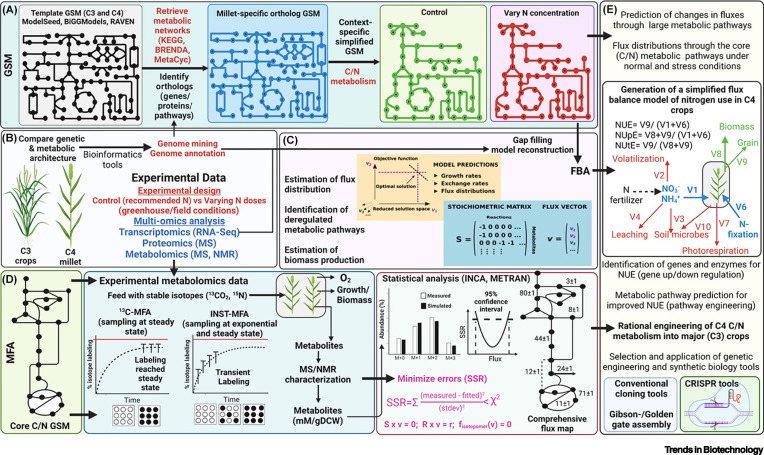
How a century of ammonia synthesis changed the world.
Nat. Geosci. 2008; 1: 636-639
Reactive nitrogen in the environment and its effect on climate change.
Curr. Opin. Environ. Sustain. 2011; 3: 281-290
Mainstreaming orphan millets for advancing climate smart agriculture to secure nutrition and health.
Front. Plant Sci. 2022; 13902536
Systems metabolic engineering strategies: integrating systems and synthetic biology with metabolic engineering.
Trends Biotechnol. 2019; 37: 817-837
Orphan crops and their wild relatives in the genomic era.
Mol. Plant. 2021; 14: 27-39
A difference in N use efficiency in C3 and C4 plants and its implications in adaptation and evolution.
Crop Sci. 1978; 18: 93-98
Differences between maize and rice in N-use efficiency for photosynthesis and protein allocation.
Plant Cell Physiol. 2003; 44: 952-956
Carbon dioxide diffusion inside leaves.
Plant Physiol. 1996; 110: 339-346
C4 photosynthesis and water stress.
Ann. Bot. 2009; 103: 635-644
Identification of biomarker for determining genotypic potential of nitrogen-use-efficiency and optimization of the nitrogen inputs in crop plants.
J. Crop. Sci. Biotechnol. 2009; 12: 183-194
Influence of nitrogen on the expression of TaDof1 transcription factor in wheat and its relationship with photosynthetic and ammonium assimilating efficiency.
Mol. Biol. Rep. 2009; 36: 2209-2220
Metabolic flux analysis of secondary metabolism in plants.
Metab. Eng. Commun. 2020; 10e00123
SiMYB19 from foxtail millet (Setaria italica) confers transgenic rice tolerance to high salt stress in the field.
Int. J. Mol. Sci. 2022; 23: 756
Current status and applications of genome-scale metabolic models.
Genome Biol. 2019; 20: 121
Metabolic flux analysis in plants: coping with complexity.
Plant Cell Environ. 2009; 32: 1241-1257
Mapping photoautotrophic metabolism with isotopically nonstationary 13C flux analysis.
Metab. Eng. 2011; 13: 656-665
Applications of stable isotope-based metabolomics and fluxomics toward synthetic biology of cyanobacteria.
Wiley Interdiscip. Rev. Syst. Biol. Med. 2020; 12e1472
Isotopically nonstationary 13C flux analysis of changes in Arabidopsis thaliana leaf metabolism due to high light acclimation.
Proc. Natl. Acad. Sci. U. S. A. 2014; 111: 16967-16972
INCA: a computational platform for isotopically non-stationary metabolic flux analysis.
Bioinformatics. 2014; 30: 1333-1335
A mass and charge balanced metabolic model of Setaria viridis revealed mechanisms of proton balancing in C4 plants.
BMC Bioinformatics. 2019; 20: 357
Systems biology for crop improvement.
Plant Genome. 2021; 14e20098
The putative glutamate receptor 1.1 (AtGLR1.1) functions as a regulator of carbon and nitrogen metabolism in Arabidopsis thaliana.
Proc. Natl. Acad. Sci. U. S. A. 2003; 100: 6872
Systematic comparison of C3 and C4 plants based on metabolic network analysis.
BMC Syst. Biol. 2012; 6: S9
Evolution of C4 photosynthesis predicted by constraint-based modelling.
eLife. 2019; 8e49305
CRISPR/Cas genome editing and precision plant breeding in agriculture.
Annu. Rev. Plant Biol. 2019; 70: 667-697
Metabolic engineering with Dof1 transcription factor in plants: improved nitrogen assimilation and growth under low-nitrogen conditions.
Proc. Natl. Acad. Sci. U. S. A. 2004; 101: 7833-7838
Fluctuation of Dof1/Dof2 expression ratio under the influence of varying nitrogen and light conditions: involvement in differential regulation of nitrogen metabolism in two genotypes of finger millet (Eleusine coracana L.).
Gene. 2014; 546: 327-335
Introduction of the ZmDof1 gene into rice enhances carbon and nitrogen assimilation under low-nitrogen conditions.
Plant Biotechnol. J. 2011; 9: 826-837
A PII-like protein in Arabidopsis: putative role in nitrogen sensing.
Proc. Natl. Acad. Sci. U. S. A. 1998; 95: 13965-13970
Designing future crops: challenges and strategies for sustainable agriculture.
Plant J. 2021; 105: 1165-1178
From plant metabolic engineering to plant synthetic biology: the evolution of the design/build/test/learn cycle.
Plant Sci. 2018; 273: 3-12
Common principles and best practices for engineering microbiomes.
Nat. Rev. Microbiol. 2019; 17: 725-741
Plant synthetic metabolic engineering for enhancing crop nutritional quality.
Plant Commun. 2020; 1100017
An automated Design-Build-Test-Learn pipeline for enhanced microbial production of fine chemicals.
Commun. Biol. 2018; 11: 66
Challenges and approaches to crop improvement through C3-to-C4 engineering.
Front. Plant Sci. 2021; 12: 1851
Recent advances in carbon and nitrogen metabolism in C3 plants.
Int. J. Mol. Sci. 2021; 22: 318
A balancing act: how plants integrate nitrogen and water signals.
J. Exp. Bot. 2020; 71: 4442-4451
Carbon/nitrogen homeostasis control in cyanobacteria.
FEMS Microbiol. Rev. 2020; 44: 33-53
From cyanobacteria to Archaeplastida: new evolutionary insights into PII signalling in the plant kingdom.
New Phytol. 2020; 227: 722-731
New views on PII signaling: from nitrogen sensing to global metabolic control.
Trends Microbiol. 2022; 30: 722-735
Tuning the in vitro sensing and signaling properties of cyanobacterial PII protein by mutation of key residues.
Sci. Rep. 2019; 9: 18985
The PII signaling protein from red algae represents an evolutionary link between cyanobacterial and Chloroplastida PII proteins.
Sci. Rep. 2018; 8: 790
PII-like signaling protein SbtB links cAMP sensing with cyanobacterial inorganic carbon response.
Proc. Natl. Acad. Sci. U. S. A. 2018; 115: e4861-e4869
Carbon signaling protein SbtB possesses redox-regulated apyrase activity to facilitate regulation of bicarbonate transporter SbtA.
bioRxiv. 2022; (Published online June 21, 2022. https://doi.org/10.1101/2022.05.18.492403)
The impact of the cyanobacterial carbon-regulator protein SbtB and of the second messengers cAMP and c-di-AMP on CO2-dependent gene expression.
New Phytol. 2022; 234: 1801-1816
Diurnal metabolic control in cyanobacteria requires perception of second messenger signaling molecule c-di-AMP by the carbon control protein SbtB.
Sci. Adv. 2021; 7: 568
Functional and structural characterization of PII-like protein CutA does not support involvement in heavy metal tolerance and hints at a small-molecule carrying/signaling role.
FEBS J. 2021; 288: 1142-1162
Structures of cyanobacterial bicarbonate transporter SbtA and its complex with PII-like SbtB.
Cell Discov. 2021; 7: 63
The novel PII-interactor PirC identifies phosphoglycerate mutase as key control point of carbon storage metabolism in cyanobacteria.
Proc. Natl. Acad. Sci. U. S. A. 2021; 118e2019988118
Phosphoenolpyruvate carboxylase from the cyanobacterium Synechocystis sp. PCC 6803 is under global metabolic control by PII signaling.
Mol. Microbiol. 2020; 114: 292-307
Interaction of the nitrogen regulatory protein GlnB (PII) with biotin carboxyl carrier protein (BCCP) controls acetyl-CoA levels in the cyanobacterium Synechocystis sp. PCC 6803.
Front. Microbiol. 2016; 7: 1700
Chloroplast acetyl-CoA carboxylase activity is 2-oxoglutarate-regulated by interaction of PII with the biotin carboxyl carrier subunit.
Proc. Natl. Acad. Sci. U. S. A. 2010; 107: 502-507
Interaction of N-acetyl-l-glutamate kinase with the PII signal transducer in the non-photosynthetic alga Polytomella parva: co-evolution towards a hetero-oligomeric enzyme.
FEBS J. 2020; 287: 465-482
The novel PII-interacting protein PirA controls flux into the cyanobacterial ornithine–ammonia cycle.
mBio. 2021; 12e00229-21
Regulation of Herbaspirillum seropedicae NifA by the GlnK PII signal transduction protein is mediated by effectors binding to allosteric sites.
Biochim. Biophys. Acta Proteins Proteom. 2020; 1868140348
Disrupting hierarchical control of nitrogen fixation enables carbon-dependent regulation of ammonia excretion in soil diazotrophs.
PLoS Genet. 2021; 17e1009617
Metabolic regulation of nitrogenase activity in Rhodospirillum rubrum: the role of PII proteins and membrane sequestration.
Biol. Nitrogen Fixat. 2015; 1–2: 131-138
Crystal structure of the GlnZ–DraG complex reveals a different form of PII-target interaction.
Proc. Natl. Acad. Sci. U. S. A. 2011; 108: 18972-18976
C4GEM, a genome-scale metabolic model to study C4 plant metabolism.
Plant Physiol. 2010; 154: 1871-1885
Zea mays iRS1563: a comprehensive genome-scale metabolic reconstruction of maize metabolism.
PLoS One. 2011; 6e21784
Assessing the metabolic impact of nitrogen availability using a compartmentalized maize leaf genome-scale model.
Plant Physiol. 2014; 166: 1659-1674
Genome-scale metabolic reconstruction of the symbiosis between a leguminous plant and a nitrogen-fixing bacterium.
Nat. Commun. 2020; 11: 2574
A genome-scale metabolic model of soybean (Glycine max) highlights metabolic fluxes in seedlings.
Plant Physiol. 2019; 180: 1912-1929
Flux balance analysis of barley seeds: a computational approach to study systemic properties of central metabolism.
Plant Physiol. 2009; 149: 585-598
Metabolic control of nitrogen fixation in rhizobium–legume symbioses.
Sci. Adv. 2021; 7eabh2433
Increasing yield potential through manipulating of an ARE1 ortholog related to nitrogen use efficiency in wheat by CRISPR/Cas9.
J. Integr. Plant Biol. 2021; 63: 1649-1663
Improving nitrogen use efficiency by manipulating nitrate remobilization in plants.
Nat. Plants. 2020; 69: 1126-1135
pOsNAR2.1: OsNAR2.1 expression enhances nitrogen uptake efficiency and grain yield in transgenic rice plants.
Plant Biotechnol. J. 2017; 15: 1273
Nitrogen-dependent posttranscriptional regulation of the ammonium transporter AtAMT1;1.
Plant Physiol. 2007; 143: 732-744
Arabidopsis LHT1 is a high-affinity transporter for cellular amino acid uptake in both root epidermis and leaf mesophyll.
Plant Cell. 2006; 18: 1931-1946
Over-expression of STP13, a hexose transporter, improves plant growth and nitrogen use in Arabidopsis thaliana seedlings.
Plant Cell Environ. 2009; 32: 271-285
Improving plant nitrogen use efficiency through alteration of amino acid transport processes.
Plant Physiol. 2017; 175: 235-247
Genetic engineering of improved nitrogen use efficiency in rice by the tissue-specific expression of alanine aminotransferase.
Plant Biotechnol. J. 2008; 6: 722-732
Improving nitrogen use efficiency through overexpression of alanine aminotransferase in rice, wheat, and barley.
Front. Plant Sci. 2021; 12628521
OsASN1 overexpression in rice increases grain protein content and yield under nitrogen-limiting conditions.
Plant Cell Physiol. 2020; 61: 1309-1320
Increased glutamine synthetase by overexpression of TaGS1 improves grain yield and nitrogen use efficiency in rice.
Plant Physiol. Biochem. 2021; 169: 259-268
The ferredoxin-dependent glutamate synthase (OsFd-GOGAT) participates in leaf senescence and the nitrogen remobilization in rice.
Mol. Gen. Genomics. 2017; 292: 385-395
Heterotrimeric G proteins regulate nitrogen-use efficiency in rice.
Nat. Genet. 2014; 46: 652-656
Identification of nitrogen use efficiency genes in barley: searching for QTLs controlling complex physiological traits.
Front. Plant Sci. 2016; 7: 1587
Increased nitrogen-use efficiency in transgenic rice plants over-expressing a nitrogen-responsive early nodulin gene identified from rice expression profiling.
Plant Cell Environ. 2009; 32: 1749-1760
Cytokinin-dependent improvement in transgenic PSARK::IPT tobacco under nitrogen deficiency.
J. Agric. Food Chem. 2011; 59: 10491-10495
Soybean SAT1 (symbiotic ammonium transporter 1) encodes a bHLH transcription factor involved in nodule growth and NH4+ transport.
Proc. Natl. Acad. Sci. U. S. A. 2014; 111: 4814-4819
Plant nuclear factor Y (NF-Y) B subunits confer drought tolerance and lead to improved corn yields on water-limited acres.
Proc. Natl. Acad. Sci. U. S. A. 2007; 104: 16450-16455
The nitrate-inducible NAC transcription factor tanac2-5a controls nitrate response and increases wheat yield.
Plant Physiol. 2015; 169: 1991
A wheat transcription factor positively sets seed vigour by regulating the grain nitrate signal.
New Phytol. 2020; 225: 1667-1680
- SEO Powered Content & PR Distribution. Get Amplified Today.
- Platoblockchain. Web3 Metaverse Intelligence. Knowledge Amplified. Access Here.
- Source: https://www.cell.com/trends/biotechnology/fulltext/S0167-7799(22)00279-7?rss=yes
- 1
- 2022
- a
- Act
- activity
- advances
- agriculture
- allocation
- analysis
- and
- applications
- approach
- approaches
- article
- authors
- Automated
- availability
- Balance
- based
- BEST
- best practices
- between
- biology
- Bot
- Camp
- carbon
- central
- Century
- challenges
- change
- Changes
- charge
- Climate
- Climate change
- comparison
- complex
- complexity
- comprehensive
- conditions
- content
- control
- controlling
- controls
- crop
- crops
- determining
- difference
- different
- Drought
- Early
- effect
- efficiency
- enables
- Engineering
- enhanced
- enhancing
- Environment
- Era
- evolution
- facilitate
- field
- fine
- FLUX
- food
- form
- from
- functions
- future
- Gen
- genomics
- Global
- Growth
- Health
- High
- highlights
- hints
- How
- HTTPS
- identified
- identifies
- Impact
- implications
- improved
- improvement
- in
- Increases
- influence
- insights
- integrate
- Integrating
- interaction
- involved
- involvement
- Key
- Kingdom
- lead
- levels
- light
- LINK
- links
- List
- manipulating
- Mass
- Messenger
- metal
- Millet
- model
- modelling
- models
- molecule
- network
- New
- novel
- nuclear
- ONE
- online
- optimization
- perception
- Photosynthesis
- pipeline
- plants
- platform
- plato
- Plato Data Intelligence
- PlatoData
- Point
- potential
- practices
- Precision
- predicted
- principles
- processes
- Production
- profiling
- properties
- Protein
- Proteins
- quality
- ratio
- Red
- Regulate
- Regulation
- regulator
- regulatory
- related
- relationship
- relatives
- represents
- requires
- response
- Revealed
- Reveals
- Rice
- Role
- root
- salt
- SCI
- searching
- Second
- secondary
- secure
- seed
- seeds
- sequestration
- Sets
- Signal
- signals
- Sites
- smart
- stable
- Status
- storage
- strategies
- stress
- structural
- structure
- Study
- support
- sustainable
- Symbiotic
- synthetic
- systemic
- Systems
- The
- the world
- their
- Through
- to
- tolerance
- toward
- towards
- transport
- under
- use
- View
- views
- W
- Water
- Wild
- world
- X
- Yield
- yields
- zephyrnet